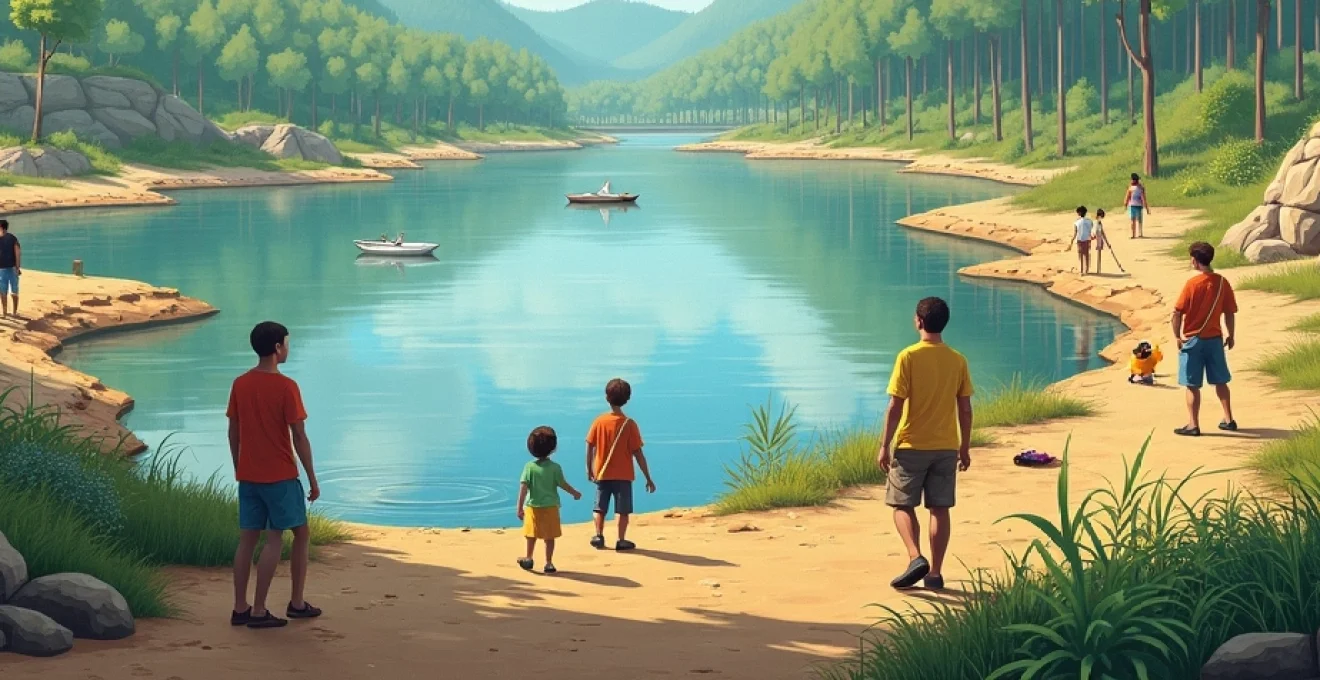
The restoration of damaged natural ecosystems is a critical endeavor in our fight against biodiversity loss and climate change. As human activities continue to degrade habitats worldwide, the need for effective restoration strategies has never been more pressing. Ecosystem restoration involves a complex interplay of ecological processes, requiring a deep understanding of natural systems and innovative approaches to revitalize degraded environments. From forests to wetlands, grasslands to coral reefs, each ecosystem presents unique challenges and opportunities for recovery.
Successful restoration not only revives biodiversity but also enhances ecosystem services vital for human well-being, such as clean water provision, carbon sequestration, and natural disaster mitigation. By employing a combination of passive and active restoration techniques, scientists and conservationists are working tirelessly to heal our planet's wounds and create resilient landscapes for future generations.
Ecosystem assessment and damage quantification techniques
Before embarking on any restoration project, it's crucial to conduct a thorough assessment of the damaged ecosystem. This initial step provides a baseline for measuring progress and helps identify the most appropriate restoration strategies. You'll need to employ a range of techniques to quantify the extent of damage and understand the ecosystem's current state.
One key method is remote sensing, which uses satellite imagery and aerial photography to map large areas and detect changes in vegetation cover, land use, and water bodies. This technology allows you to track ecosystem degradation over time and identify priority areas for restoration. Ground-based surveys complement remote sensing data, providing detailed information on species composition, soil quality, and hydrological conditions.
Another important tool is biodiversity assessment, which involves systematic sampling of flora and fauna to determine species richness and abundance. This can include techniques such as point counts for birds, transect surveys for plants, and camera traps for elusive wildlife. By comparing these data to reference ecosystems or historical records, you can gauge the level of biodiversity loss and set realistic restoration targets.
Soil analysis is equally critical, as soil health underpins ecosystem function. You should assess physical properties like texture and structure, as well as chemical characteristics such as pH, nutrient content, and organic matter. In some cases, you may need to test for contaminants that could hinder restoration efforts. Advanced techniques like DNA metabarcoding can provide insights into soil microbial communities, which play a vital role in ecosystem recovery.
Accurate ecosystem assessment is the foundation of successful restoration. Without a clear understanding of what's been lost, it's impossible to chart a path towards recovery.
Passive restoration strategies: natural regeneration processes
Passive restoration relies on the inherent ability of ecosystems to recover naturally once stressors are removed. This approach can be highly effective and cost-efficient in many situations, particularly where the damage is not too severe and seed sources are available nearby. You'll find that passive restoration often involves creating the right conditions for natural processes to take over, rather than actively intervening in the ecosystem.
Soil seed bank activation and seedling recruitment
One of the most powerful tools in passive restoration is the soil seed bank—a natural repository of dormant seeds that can remain viable for years or even decades. By creating favorable conditions for germination, you can activate this hidden potential. This might involve reducing competition from invasive species, improving soil moisture retention, or altering light conditions to break seed dormancy.
Seedling recruitment is the next crucial step in this process. Once seeds germinate, young plants need to establish themselves successfully. You can enhance recruitment by protecting seedlings from herbivores, reducing competition from faster-growing species, and ensuring adequate water and nutrient availability during the critical early growth stages.
Mycorrhizal networks in forest recovery
In forest ecosystems, mycorrhizal fungi play a vital role in restoration by forming symbiotic relationships with plant roots. These fungi create vast underground networks that facilitate nutrient exchange between plants and improve soil structure. By preserving existing mycorrhizal networks or inoculating soil with appropriate fungal species, you can significantly accelerate forest recovery.
The wood wide web, as these fungal networks are sometimes called, not only supports individual trees but also enables communication and resource sharing between different plant species. This interconnectedness is crucial for building resilient forest ecosystems that can withstand future disturbances.
Hydrological regime restoration for wetland ecosystems
Wetland restoration often hinges on reestablishing natural hydrological patterns. This might involve removing drainage structures, reconnecting floodplains to rivers, or managing water levels to mimic natural fluctuations. By restoring the water regime, you create conditions that favor wetland plant species and support the return of aquatic and semi-aquatic wildlife.
It's important to consider the broader watershed context when restoring wetland hydrology. Upstream land use changes can have significant impacts on water quality and quantity, so you may need to work with landowners and policymakers to address these larger-scale issues.
Successional dynamics in grassland rehabilitation
Grassland ecosystems often demonstrate remarkable resilience when given the opportunity to recover naturally. Understanding successional dynamics is key to successful passive restoration in these habitats. Early successional species, often annual plants, can quickly colonize disturbed areas, stabilizing the soil and creating conditions for later successional species to establish.
Fire can be a powerful tool in grassland restoration, mimicking natural disturbance regimes that many grassland species have evolved with. Carefully managed prescribed burns can help control woody encroachment, recycle nutrients, and stimulate the germination of fire-adapted plant species.
Active restoration interventions: reintroduction and revegetation
While passive restoration can be effective in many cases, some ecosystems require more direct intervention to recover. Active restoration involves deliberately reintroducing species and manipulating environmental conditions to accelerate ecosystem recovery. This approach is often necessary in severely degraded areas or where natural regeneration is hindered by factors such as invasive species or altered soil conditions.
Native species selection criteria for ecosystem compatibility
Choosing the right species for reintroduction is crucial for restoration success. You should prioritize native species that are well-adapted to local conditions and play important ecological roles. Consider factors such as:
- Habitat requirements and niche specificity
- Functional traits that contribute to ecosystem processes
- Interactions with other species, including pollinators and seed dispersers
- Resilience to climate change and other potential future stressors
- Availability of source populations or propagation material
It's often beneficial to use a diverse mix of species that represent different functional groups and life forms. This approach helps build ecosystem resilience and enhances the range of ecosystem services provided.
Propagation techniques for keystone plant species
Successful revegetation often depends on your ability to propagate and establish keystone plant species. These are species that have a disproportionate impact on ecosystem function relative to their abundance. Propagation techniques vary depending on the species and ecosystem type, but may include:
- Seed collection and storage
- Nursery cultivation of seedlings or cuttings
- Direct seeding techniques such as hydro-seeding or seed bombing
- Vegetative propagation methods like layering or division
- Advanced techniques such as tissue culture for rare or difficult-to-propagate species
Ensuring genetic diversity in propagated plants is crucial for long-term ecosystem health. You should collect propagation material from multiple source populations when possible to maintain adaptive potential.
Fauna reintroduction: ecological role of megafauna
While plant communities form the foundation of most terrestrial ecosystems, animal reintroductions can play a critical role in restoration. This is particularly true for megafauna—large animals that often act as ecosystem engineers, shaping habitats through their feeding and movement patterns. Examples include elephants in African savannas or bison in North American prairies.
Reintroducing megafauna can have cascading effects throughout the ecosystem. These animals can help disperse seeds, create habitat heterogeneity, and regulate plant communities through grazing or browsing. However, megafauna reintroductions require careful planning and management to ensure the safety of both animals and human communities.
Assisted migration in climate change adaptation
As climate change alters environmental conditions at an unprecedented rate, some species may be unable to adapt or migrate quickly enough to survive. Assisted migration—the deliberate movement of species to new areas outside their historical range—is a controversial but potentially necessary strategy for preserving biodiversity in the face of climate change.
When considering assisted migration, you must carefully weigh the potential benefits against the risks of introducing species to new ecosystems. Thorough ecological assessments and modeling of future climate scenarios are essential to inform these decisions.
Bioengineering approaches for ecosystem stabilization
Bioengineering combines ecological principles with engineering techniques to create sustainable solutions for ecosystem restoration. These approaches often focus on stabilizing degraded landscapes and creating favorable conditions for natural recovery processes to take hold.
Erosion control using living systems
Soil erosion is a major challenge in many degraded ecosystems, particularly on steep slopes or in areas with high rainfall. Bioengineering techniques for erosion control use living plants and plant materials to stabilize soil and reduce runoff. Some effective methods include:
- Contour wattling: Installing bundles of live branches across slopes
- Live staking: Planting cuttings of fast-growing tree or shrub species directly into the soil
- Brush layering: Alternating layers of soil and live branches on steep slopes
- Vegetated gabions: Wire baskets filled with rocks and live plant material
- Geotextiles made from biodegradable materials combined with seeding
These techniques not only provide immediate stabilization but also establish vegetation that will continue to protect the soil as it grows and develops root systems.
Phytoremediation for contaminated soil restoration
In ecosystems contaminated by pollutants, phytoremediation can be an effective and environmentally friendly restoration strategy. This approach uses plants to remove, degrade, or stabilize contaminants in soil or water. Different phytoremediation mechanisms include:
- Phytoextraction: Plants absorb contaminants from soil and accumulate them in harvestable biomass
- Phytostabilization: Plants reduce the mobility and bioavailability of contaminants in soil
- Phytodegradation: Plants break down contaminants through metabolic processes
- Rhizofiltration: Plant roots filter contaminants from water
Selecting the right plant species for phytoremediation is crucial and depends on the type of contaminant, soil conditions, and climate. Some plants, known as hyperaccumulators, have an exceptional ability to take up specific pollutants and are particularly valuable for this purpose.
Constructed wetlands for water quality improvement
Constructed wetlands are engineered systems that mimic the water purification processes of natural wetlands. These systems can be highly effective in treating various types of wastewater, including agricultural runoff, urban stormwater, and even some industrial effluents. The design of constructed wetlands typically incorporates:
- A substrate of soil or gravel to support plant growth and microbial communities
- Carefully selected wetland plants that tolerate saturated conditions
- Hydraulic structures to control water flow and retention time
- Microbial communities that break down pollutants
As water flows through the constructed wetland, a combination of physical, chemical, and biological processes removes contaminants. This not only improves water quality but also creates valuable habitat for wildlife and can enhance landscape aesthetics.
Monitoring and adaptive management in restoration projects
Ecosystem restoration is not a one-time intervention but an ongoing process that requires careful monitoring and adaptive management. You need to track progress towards restoration goals, identify emerging challenges, and adjust strategies as needed. Effective monitoring programs typically include:
- Regular surveys of plant and animal communities
- Assessments of soil and water quality
- Monitoring of key ecosystem processes such as nutrient cycling
- Evaluation of ecosystem services provision
- Documentation of human interactions with the restored ecosystem
Data collected through monitoring should be used to inform adaptive management decisions. This might involve adjusting planting strategies, modifying water management practices, or implementing additional interventions to address unexpected challenges. By maintaining flexibility and responsiveness, you can increase the likelihood of long-term restoration success.
Adaptive management is the key to navigating the complexities of ecosystem restoration. Nature is dynamic, and our approaches must be equally flexible and responsive.
Policy frameworks and socioeconomic aspects of ecosystem restoration
Successful ecosystem restoration requires more than just ecological expertise—it also depends on supportive policy frameworks and consideration of socioeconomic factors. You need to engage with a wide range of stakeholders, including local communities, policymakers, and industries that may be impacted by restoration activities.
Policy support for ecosystem restoration can take many forms, including:
- Legal protection for restored areas
- Financial incentives for landowners to undertake restoration
- Integration of restoration goals into land-use planning and development policies
- Support for research and capacity building in restoration science
- Mechanisms to value and compensate for ecosystem services
Addressing socioeconomic aspects of restoration is crucial for long-term success. This might involve creating alternative livelihoods for communities that previously depended on unsustainable resource extraction, or developing ecotourism opportunities in restored areas. By demonstrating the tangible benefits of healthy ecosystems, you can build local support and ensure the sustainability of restoration efforts.
International initiatives like the UN Decade on Ecosystem Restoration (2021-2030) are helping to galvanize global action and provide frameworks for coordinated restoration efforts. These platforms offer opportunities for knowledge sharing, resource mobilization, and scaling up successful restoration approaches.
As you embark on ecosystem restoration projects, remember that each site presents unique challenges and opportunities. By combining scientific rigor with creativity and adaptability, and by engaging meaningfully with local communities and policymakers, you can make significant contributions to healing our planet's ecosystems and securing a more sustainable future for all.