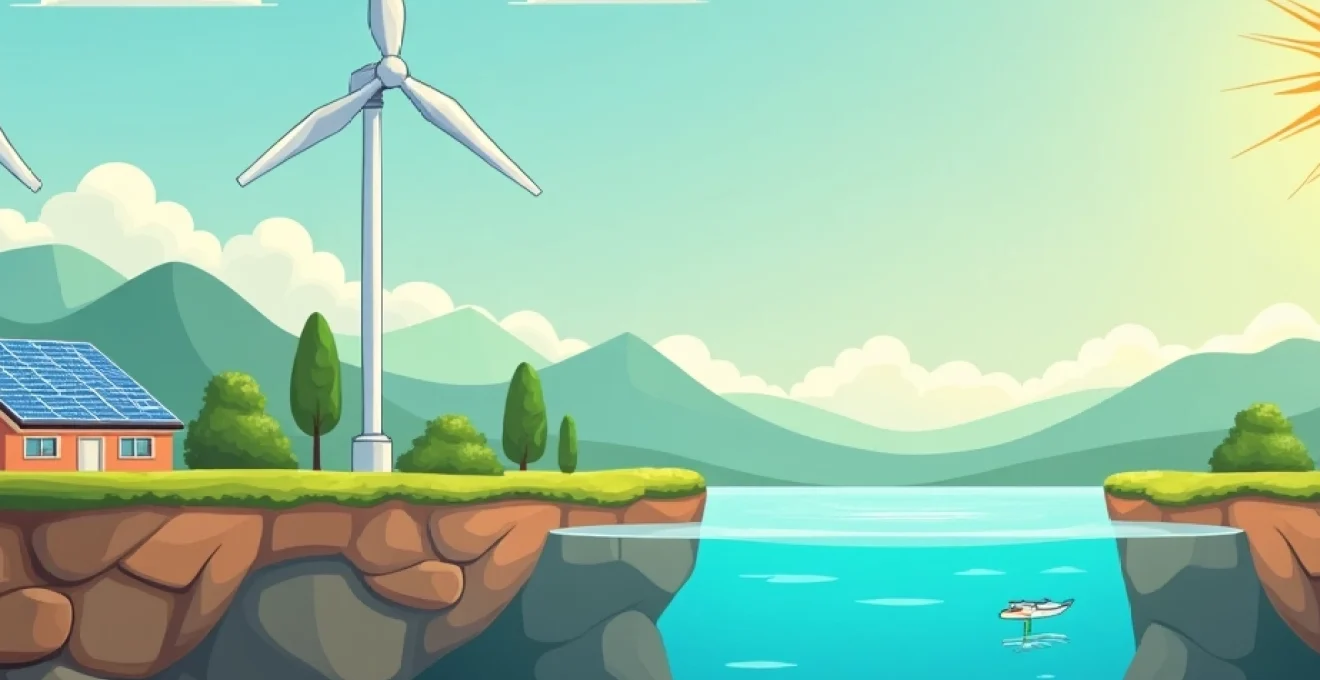
Renewable resources are natural assets that can replenish themselves over time, offering a sustainable alternative to finite fossil fuels. As the world grapples with climate change and energy security concerns, these resources have become increasingly vital. From solar and wind power to hydroelectric and geothermal energy, renewable sources are reshaping our approach to power generation and consumption. Their importance extends beyond environmental benefits, encompassing economic advantages and technological innovations that are driving a global shift towards cleaner, more sustainable energy systems.
Types of renewable resources: solar, wind, hydro, and geothermal
Renewable resources encompass a diverse range of naturally replenishing energy sources. Solar power harnesses the sun's radiation, while wind energy captures the kinetic force of moving air. Hydroelectric power taps into the energy of flowing water, and geothermal systems utilize heat from the Earth's core. Each of these resources offers unique advantages and applications, contributing to a more diversified and resilient energy landscape.
The versatility of renewable resources allows for their implementation across various scales and geographical locations. From large utility-scale installations to small residential systems, these technologies are adaptable to different energy needs and environmental conditions. As research and development continue to advance, the efficiency and accessibility of renewable resources continue to improve, making them increasingly competitive with traditional fossil fuel-based energy sources.
Photovoltaic technology: advancements in solar energy capture
Photovoltaic (PV) technology has undergone remarkable advancements in recent years, dramatically improving the efficiency and cost-effectiveness of solar energy capture. These innovations have propelled solar power to the forefront of renewable energy adoption, with global installations increasing exponentially. The evolution of PV technology encompasses various cell types and manufacturing processes, each offering distinct advantages for different applications.
Monocrystalline vs. polycrystalline silicon cells
Silicon-based solar cells dominate the market, with two primary types: monocrystalline and polycrystalline. Monocrystalline cells, made from a single crystal structure, offer higher efficiency and a sleek appearance. They typically convert 20-22% of sunlight into electricity. Polycrystalline cells, composed of multiple silicon fragments, are less expensive to produce but slightly less efficient, with conversion rates around 15-17%. The choice between these types often depends on specific project requirements, balancing cost and performance considerations.
Thin-film solar panels and CIGS technology
Thin-film solar technology represents a departure from traditional silicon-based cells. These panels are lightweight, flexible, and can be integrated into various surfaces. Copper Indium Gallium Selenide (CIGS) is a promising thin-film technology, offering efficiency rates approaching those of silicon cells while using less material. CIGS panels are particularly well-suited for applications where weight and flexibility are crucial, such as building-integrated photovoltaics or portable solar chargers.
Perovskite solar cells: next-generation efficiency
Perovskite solar cells have emerged as a groundbreaking technology in photovoltaics. These cells use a unique crystal structure that can be engineered to absorb different parts of the light spectrum efficiently. Research has shown perovskite cells achieving efficiency rates over 25% in laboratory settings, surpassing many commercial silicon cells. The potential for low-cost manufacturing and high efficiency makes perovskite technology a promising candidate for the next generation of solar panels.
Concentrated solar power (CSP) systems
Concentrated Solar Power systems take a different approach to harnessing solar energy. Instead of directly converting light to electricity, CSP uses mirrors or lenses to focus sunlight onto a small area, generating intense heat. This heat is then used to drive a turbine or engine to produce electricity. CSP systems can incorporate thermal storage, allowing for power generation even when the sun isn't shining. This ability to provide dispatchable power makes CSP an attractive option for utility-scale projects in regions with high direct sunlight.
Wind energy: onshore and offshore turbine innovations
Wind energy has seen remarkable growth and technological advancement in recent years. Innovations in turbine design, materials, and control systems have significantly increased energy output while reducing costs. Both onshore and offshore wind installations play crucial roles in the renewable energy mix, each with its own set of technological challenges and opportunities.
Horizontal-axis vs. vertical-axis wind turbines
Horizontal-axis wind turbines (HAWTs) are the most common type, characterized by their propeller-like blades rotating around a horizontal axis. These turbines are highly efficient at capturing wind energy, especially in areas with consistent wind directions. Vertical-axis wind turbines (VAWTs), on the other hand, rotate around a vertical axis and can capture wind from any direction without needing to reorient. While less common, VAWTs have advantages in urban environments or areas with turbulent wind patterns.
Floating offshore wind farms: Hywind Scotland project
The Hywind Scotland project represents a significant milestone in offshore wind technology. As the world's first floating wind farm, it demonstrates the feasibility of installing wind turbines in deep waters where traditional fixed-bottom foundations are impractical. Floating wind farms open up vast new areas for wind energy development, particularly in regions with steep coastal shelves. The success of Hywind Scotland has spurred interest in floating wind technology worldwide, with several countries now planning large-scale floating wind projects.
Airborne wind energy systems: Makani's energy kite
Airborne wind energy systems represent an innovative approach to harnessing wind power at higher altitudes where winds are stronger and more consistent. Makani, a former subsidiary of Alphabet, developed an energy kite that flies in large circles while tethered to the ground. The kite's movement drives generators to produce electricity. While Makani's project has ended, the concept of airborne wind energy continues to attract research and development efforts, promising to tap into wind resources inaccessible to traditional turbines.
Small-scale wind: residential micro-turbines
Residential micro-turbines are bringing wind energy to the individual homeowner level. These small-scale turbines are designed for urban or suburban environments, often incorporating vertical-axis designs to handle turbulent wind conditions. While not as efficient as large-scale turbines, micro-turbines can supplement a home's energy supply, particularly when combined with solar panels in a hybrid renewable energy system. Advances in materials and design are making these systems more affordable and easier to install, broadening their appeal to homeowners interested in generating their own clean energy.
Hydroelectric power: large-scale dams to micro-hydro systems
Hydroelectric power remains one of the most established and widely used forms of renewable energy. It harnesses the energy of flowing water to generate electricity, offering a reliable and flexible power source. The scale of hydroelectric installations ranges from massive dams that can power entire regions to small micro-hydro systems suitable for individual buildings or remote communities.
Large-scale hydroelectric dams, while controversial due to their environmental impact, continue to play a significant role in many countries' energy portfolios. These projects can generate enormous amounts of power and often serve multiple purposes, including flood control and water management. However, the focus has increasingly shifted towards more environmentally friendly hydroelectric solutions.
Run-of-river hydroelectric systems have gained popularity as a less disruptive alternative to large dams. These installations divert a portion of a river's flow through turbines before returning it to the main channel, minimizing the need for large reservoirs. This approach reduces the ecological impact while still providing substantial power generation capabilities.
At the smallest scale, micro-hydro systems are emerging as a viable option for decentralized power generation. These systems can be installed on small streams or even in municipal water systems, providing localized power without the need for extensive infrastructure. Advances in turbine design and control systems have made micro-hydro increasingly efficient and cost-effective, opening up new possibilities for hydroelectric power in areas previously considered unsuitable.
Geothermal energy: tapping earth's core heat
Geothermal energy harnesses the heat stored beneath the Earth's surface, offering a consistent and renewable power source. Unlike solar or wind, geothermal energy is available 24/7, making it an attractive option for baseload power generation. The technology to tap into this resource has evolved significantly, allowing for its use in a wider range of geological settings.
Enhanced geothermal systems (EGS) technology
Enhanced Geothermal Systems (EGS) represent a breakthrough in geothermal technology, allowing for power generation in areas without naturally occurring hot water reservoirs. EGS involves creating artificial reservoirs by injecting water into hot, dry rock formations deep underground. This water is then heated by the rock and pumped back to the surface to drive turbines. EGS technology has the potential to dramatically expand the geographical range of geothermal power, making it a viable option in many more locations worldwide.
Binary cycle power plants for low-temperature resources
Binary cycle power plants have opened up new possibilities for geothermal energy production from lower-temperature resources. These systems use a secondary fluid with a lower boiling point than water, allowing for electricity generation from geothermal resources as cool as 57°C (135°F). The geothermal fluid heats the secondary fluid, which vaporizes and drives a turbine. This technology has made geothermal power generation feasible in many areas previously considered unsuitable, significantly expanding the potential for geothermal energy utilization.
Geothermal heat pumps for residential applications
Geothermal heat pumps offer a way for individual homes and buildings to tap into the Earth's constant temperature for heating and cooling. These systems circulate a fluid through underground pipes, using the Earth as a heat source in winter and a heat sink in summer. While not generating electricity, geothermal heat pumps can significantly reduce energy consumption for space conditioning. Advances in heat pump technology and installation techniques have made these systems more efficient and cost-effective, driving their adoption in residential and commercial buildings.
Environmental impact and sustainability metrics of renewable resources
While renewable resources offer significant environmental benefits compared to fossil fuels, it's crucial to assess their full environmental impact and sustainability metrics. This evaluation ensures that the transition to renewable energy sources truly contributes to a more sustainable future. Various tools and methodologies have been developed to quantify and compare the environmental performance of different energy technologies.
Life cycle assessment (LCA) of renewable technologies
Life Cycle Assessment (LCA) is a comprehensive method for evaluating the environmental impacts of renewable technologies throughout their entire lifespan. This includes raw material extraction, manufacturing, transportation, installation, operation, and end-of-life disposal or recycling. LCA studies have shown that while renewable technologies generally have lower lifetime emissions than fossil fuels, they are not entirely without impact. Factors such as the energy intensity of manufacturing processes and the sourcing of raw materials can significantly affect a technology's overall environmental footprint.
Carbon footprint comparison: renewables vs. fossil fuels
The carbon footprint of renewable energy sources is substantially lower than that of fossil fuels when considering lifecycle emissions. For example, the median lifecycle emissions for solar PV are around 41 grams of CO2 equivalent per kilowatt-hour (g CO2eq/kWh), compared to 820 g CO2eq/kWh for coal. Wind energy has even lower emissions, at approximately 11 g CO2eq/kWh. These figures demonstrate the significant potential for renewable technologies to reduce greenhouse gas emissions in the energy sector.
Land use and biodiversity considerations
The land use requirements and potential impacts on biodiversity are important considerations for renewable energy projects. Solar farms and wind installations can occupy large areas, potentially competing with agriculture or natural habitats. However, innovative approaches such as agrivoltaics, which combines solar energy production with agriculture, are addressing these concerns. For wind farms, careful siting and design can minimize impacts on wildlife, particularly birds and bats. Offshore wind farms have shown potential benefits for marine ecosystems by creating artificial reefs, demonstrating that renewable energy projects can have positive ecological impacts when properly managed.
Water consumption in renewable energy production
Water consumption varies significantly among different renewable energy technologies. Photovoltaic solar panels and wind turbines have minimal water requirements during operation, primarily for cleaning. In contrast, concentrated solar power systems and some geothermal plants can have substantial water needs for cooling. Hydroelectric power, while not consuming water in the traditional sense, can affect water availability and quality in river systems. Understanding these water use patterns is crucial for sustainable energy planning, especially in water-stressed regions.
As renewable resources continue to evolve and expand, ongoing assessment of their environmental impacts and sustainability metrics is essential. This evaluation ensures that the transition to renewable energy aligns with broader sustainability goals, maximizing benefits while minimizing potential negative consequences. By considering these factors, policymakers, industry leaders, and consumers can make informed decisions that support a truly sustainable energy future.